Dr. Laura Machuca Suarez, TECHT’s BioTECHT Principal, shares her thoughts on Microbial Corrosion to help shed light on this prevalent form of corrosion and empower managers to manage it effectively. With the right know-how and strategies, we can tackle this hidden problem, safeguarding industrial assets and ensuring their smooth operation.
“I find the world of microscopic organisms fascinating. Ever since I was in school, biology has been my favourite subject, yet I only stumbled into the world of microbes by accident later in my life”. Dr. Machuca Suarez.
Her interest in microbes then grew when she realised how essential they are for life and the great impact these tiny life forms can have on the environment.
“At first, my interest lay in bioremediation, using microbes to clean up pollution. However, my focus shifted when the oil company I was working for tasked me with investigating a problem. Metal pipes and equipment were corroding too fast, and bacteria were the prime suspects. And that’s when my journey with microbial corrosion kicked off. Fast forward two decades later and thousands of miles away from the origins of my career in my native country, I’m still grappling with the same challenge, armed with more knowledge”.
What is microbial corrosion and why does it matter?
Microbial corrosion has come to be referred to as ‘microbiologically influenced corrosion’ (MIC). In simple terms, It’s the decay of materials caused by the action of microbes. Metals, concrete, and protective paintings can all fail due to microbial action. It is a serious and costly issue that impacts industries such as oil and gas, mining, marine, and water.
MIC can lead to expensive repairs, production downtime, and major risks to safety and the environment. In 2006, bacteria caused a pipeline failure that resulted in the spill of 200,000 gallons of crude oil on the Alaska North Slope, causing significant damage to the environment and costly fines for the responsible company.
Billions of dollars are spent globally each year on MIC.
Despite its widespread impact, many companies still only react to MIC issues when they arise. Asset owners often focus on meeting immediate deadlines and saving costs instead of considering life cycle costs. As assets age, the risk of MIC grows, yet prevention investments decline. Investigations into MIC failures often point to poor management as the main contributing factor.
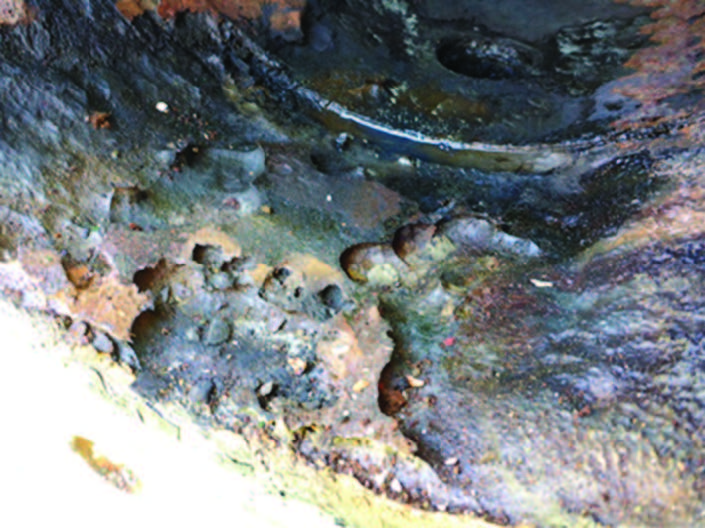
Biofilms: The Hidden Culprits Causing MIC
The process is simple, but the result is complex. As bacteria float in water, they quickly attach to a surface as soon as they sense it. They anchor firmly to it using biochemical glue forming a hardy bond that is difficult to break. They multiply and attract more bacteria. All together, they create scaffolding and network structures that encase them. The result is a cluster like a multi-cellular organism, with billions of specialized bacteria living in it, with the right tools and ability to handle dangers that come their way.
Inside biofilms, microbes cooperate, communicate, and develop defense traits, making them hard to kill. In humans, this leads to the persistence of infections, while in industry, it causes material deterioration and asset failure.
Biofilms can create aggressive environments that speed up corrosion locally, most of the time in hidden places or hard to reach. This complicates detection and control, often rendering conventional treatments ineffective. Picture this like tooth decay. As decay advances, bacteria continue their damaging journey through your teeth, reaching the pulp. Their destruction persists despite increased dental hygiene practices.
The MIC challenge lies in preventing biofilm formation and combating resistant biofilms. It should not be a fight against bacteria but against their way of living. Warfare against bacteria should target their communities, fortresses, scaffolding, bridges, and ways of communication. This should be the focus of Innovative solutions for MIC control.
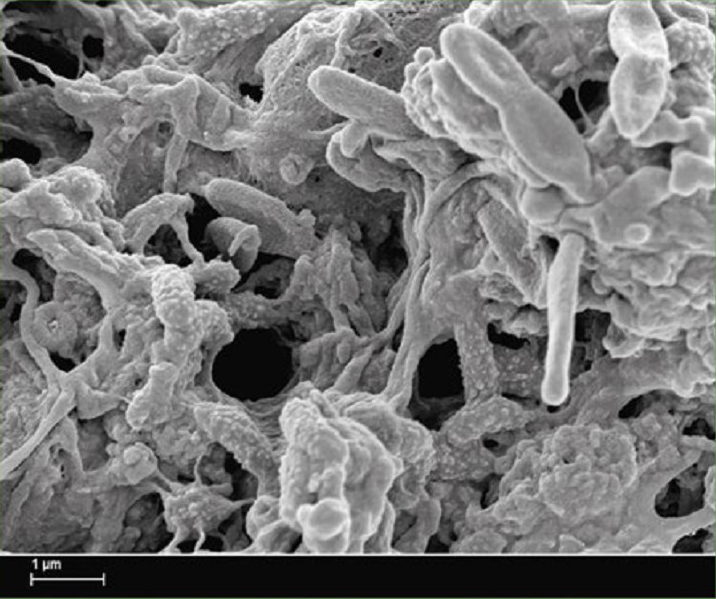
How to prevent MIC failures?
Back when I was a university Assoc. Professor, I oversaw research on microbial corrosion. After many years of studies, we got a handle on how microbial corrosion affects different materials, like plain steel and corrosion-resistant alloys, and in various locations, from oil fields to the deep sea.
The work underlined that every piece of equipment is different, and every location has its own conditions and quirks. Yet, there are common factors that lead to the onset of MIC: 1) a susceptible material, 2) the presence of microbes, and 3) the right environment that supports both corrosion and microbial activity. These are things like temperature, flow velocity, and water chemistry.
This understanding forms the foundation for assessing risks and developing successful strategies to mitigate them.
Use the Right Material
To prevent microbial corrosion, choose materials wisely. Biofilms form on nearly any material surface. Yet, their effect on corrosion depends on the material properties. For instance, in stainless steels in seawater biofilms can increase their corrosion potential significantly. This can speed up pitting, galvanic and crevice corrosion in susceptible steels. Yet, highly alloyed steels remain resistant to microbial corrosion.
When selecting materials, it also important to consider future project scenarios. Examples include potential subsea tiebacks of new oil accumulations to existing facilities or repurposing existing infrastructure for CO2 transport and storage. This can bring new challenges due to the mixing of water chemistries and commodities with different properties.
Cladding with MIC-resistant alloys offers internal protection to assets that will be exposed to high-risk scenarios where conventional treatments cannot be used. Likewise, non-metallic material options like antifouling or multifunctional coatings work well in areas with limited access that are challenging to clean and treat.
Laboratory tests can also aid in material selection and decision-making. Laboratory simulations can help engineers determine if a material is suitable for a specific application, and the MIC rates and mechanisms taking place in unusual substratum/environment scenarios.
Smart Design
Smart design can prevent microbial corrosion issues. If design ensures that water doesn’t gather and drains away well, it’s less likely for biofilms to grow. Sloping surfaces, smooth weld finishes, and the elimination of crevices, reduce the areas where microbes can harbor and grow easily.
The incorporation of accessible areas for inspection and maintenance ensures that any MIC issues can be identified and addressed promptly before they turn into big corrosion problems.
Biocides
Although not the most eco-friendly approach, implementing a chemical biocide regimen reduces the risk of microbial corrosion. The application of chemicals to kill bacteria is effective if done properly and managed carefully. When selecting biocides, it’s important to consider factors such as toxicity, degradation profile, optimal pH range, chemical incompatibilities, and efficiency against biofilms. Biocides should include additives such as bio-dispersants that target the biofilm matrix. Dosing rates and frequency should be adjusted based on performance checks.
Cleaning and Maintenance
Routine cleaning and maintenance are key in the fight against microbial corrosion. Following a cleaning schedule that helps remove biofilms and deposits is crucial to prevent MIC.
Deposits like sand, scales, mineral mixtures, and organic matter increase the risk of MIC. They can concentrate nutrients, encourage biofilm growth, and trigger under-deposit corrosion, which is a direct activator of MIC. Deposits also affect treatment efficiency since biocides and corrosion inhibitors can adsorb onto deposits. Therefore, techniques such as mechanical cleaning and flushing are essential.
A critical step is to implement a strategy to examine and repair sections of the pipeline that are difficult to inspect with an internal inspection device, particularly those at greater risk of MIC, including dead legs and areas prone to the accumulation of liquids and solids.
MIC in the Early Stages is Reversible
Early detection is key. This requires regular inspection of susceptible systems, checking for both corrosion and biofilm presence. Testing for bacteria, using smart inspection tools, and measuring corrosion rates in real time.
Nowadays, it is simple and cost-effective to use DNA methods to track bacteria more accurately. The key is to collect the right samples: biofilms. It is critical to design for and install devices that allow assessment of biofilms and place them at strategic locations (sampling points). Biofilm collection devices include corrosion coupons, discs, and side stream devices. Do not rely on data from bacteria in fluids only.
Understanding the function of the hundreds or even thousands of different species of microbes often identified in biofilms using DNA analysis can be challenging. What is the link between microbial diversity and corrosion rates? who are the key players? How do microbe type and concentration relate to MIC risk? These are questions that remain difficult to answer. This is why it is important to look at trends in data. There are limited real-time monitoring systems specific to MIC. Cost-effective tools for early detection of MIC are promising areas of technological development.
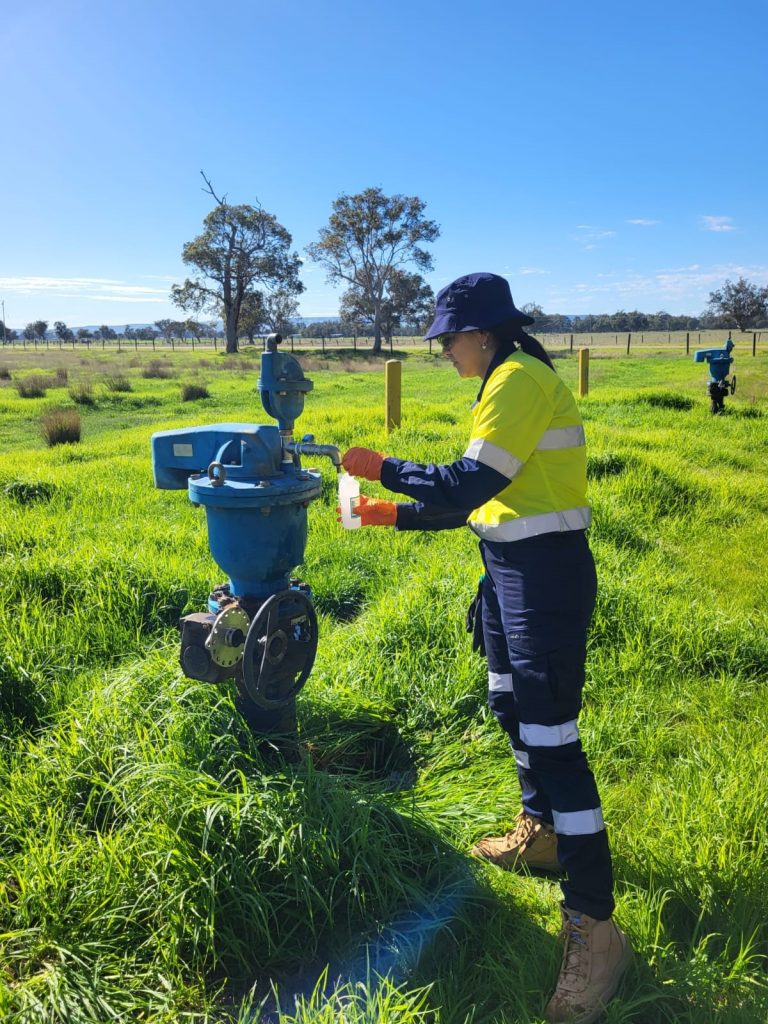
Implementing an Effective MIC Management System
Here Dr. Machuca Suarez recommendations for an effective MIC management system:
- Develop a MIC strategy and management plans for each asset.
- Identify threats and risks through the life cycle of the asset. Risk criteria include operating conditions (e.g., flow velocity and temperature), fluid composition, system microbiology, equipment design features (e.g. presence of crevice, dead legs), corrosion history, treatment options, capacity for monitoring, and inspection.
- Implement tailored mitigation measures. These should include a chemical treatment program and a physical clean-up strategy with a schedule.
- Conduct regular chemical performance checks.
- Construct a live database of information including microbial, corrosion, and inspection data.
- Deploy real-time MIC/condition monitoring systems.
- Conduct a recurrent review of the MIC strategy.
- Design and implement a monitoring program that includes biofilm testing, inspection, and corrosion rate measurements in representative locations.
- Develop a tailored management plan for high-risk areas that cannot be managed by chemical injection or inspection such as dead legs.
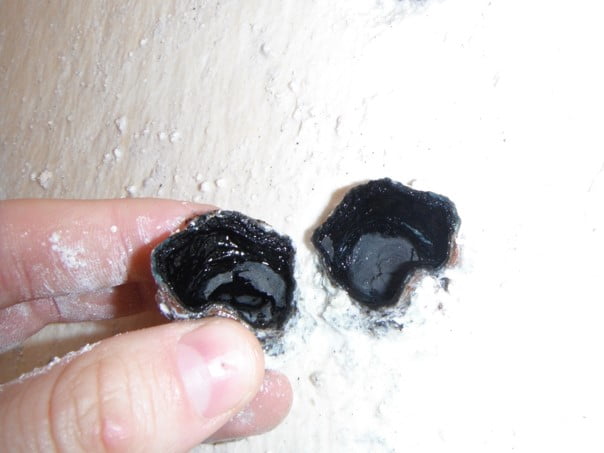
In dealing with microbial corrosion, there’s no one-size-fits-all solution. However, we can take radical action to reduce the risk of MIC and ensure equipment safety.
It begins with devising an MIC strategy and action plan for each asset. Such a strategy should include continuous assessment of threats, a regular physical cleaning schedule, using chemical treatments strategically, and a proactive plan for conducting internal inspections that assess both corrosion and biofilms. This must include a tailored strategy for inspection and management of hidden biofilm-prone areas like dead legs.
Likewise, it is essential to implement a meaningful monitoring framework. Chemical performance checks, corrosion rate measurements, and analysis of biofilms using biofilm collection devices should take place on a regular schedule.
By implementing these measures effectively, industrial facilities can greatly reduce costs and risks associated with microbial corrosion. This ensures the safety, efficiency, and durability of industrial assets, contributing to sustainable operations.
Finally, to revolutionise our approach to managing MIC we must embrace technologies that enable prediction, early detection, and real-time monitoring. We need to develop solutions to thwart biofilm formation, such as pioneering surface treatments and advanced coatings. Focusing on the biofilm matrix, we can develop more innovative and eco-friendly solutions to this pervasive MIC problem. AI promises to be a fundamental enabler of such technological solutions.
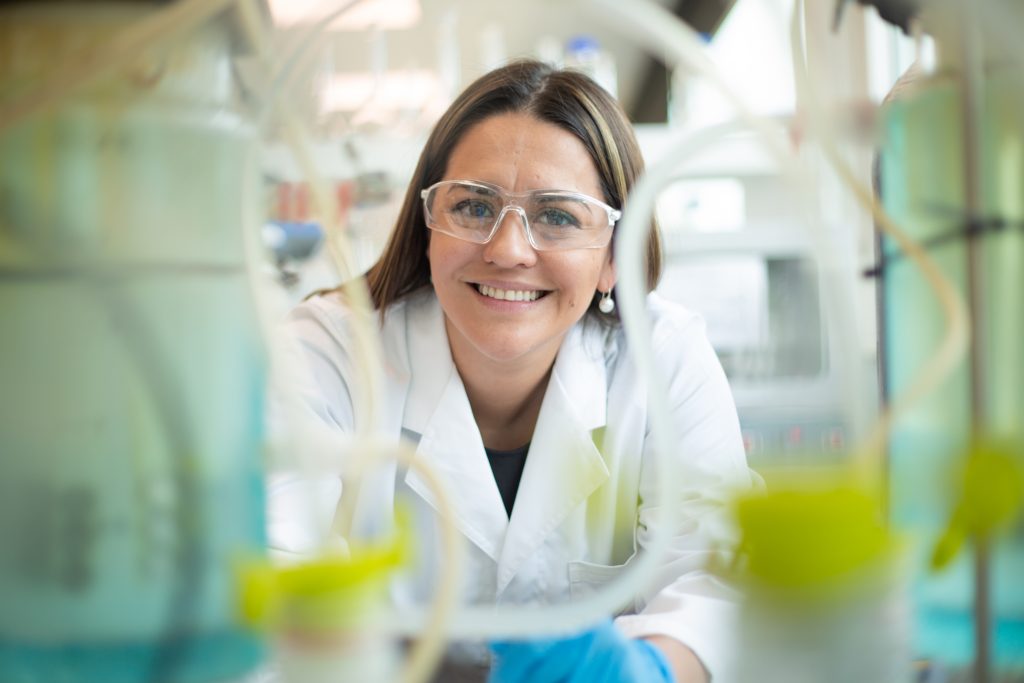
Let’s lead the charge in protecting our infrastructure and environment with smarter, innovative solutions.
The future of asset integrity and corrosion control lies in our commitment to innovation and sustainability. BioTECHT is a division of TECHT specialising in Microbiologically Influenced Corrosion (MIC).
We work closely with our clients to develop tailored and innovative microbial corrosion solutions.
Contact us to find out how we can help you.
References
- Machuca, L. L. Understanding and Addressing Microbiologically Influenced Corrosion (MIC). Corrosion and Materials, 44 (1): 88-96. 2019
- Machuca L. L. and Polomka, A. Microbiologically Influenced Corrosion in Floating Productions Systems. Microbiology Australia 39 (3). 2018. 165-169
- Machuca, L. L. et al. MIC Investigation of Stainless Steel Seal Ring Corrosion Failure in a Floating Production Storage and Offloading (FPSO) Vessel. In: R. B. Eckert & T. L. Skovhus (Eds.), Failure Analysis of Microbiologically Influenced Corrosion. (1 ed.). CRC Press. 2021
- Machuca, L. L. et al. Corrosion of carbon steel in the presence of oilfield deposit and thiosulphate-reducing bacteria in CO2 environment. Corrosion Science, 2017. 129, 16-25
- Machuca L. L., et al (2016). Evaluation of the effects of seawater ingress into 316L lined pipes on corrosion performance. Materials and Corrosion, 2014. 65 (1), 8-17.
- Machuca L.L. (2017). Microbiologically Induced Corrosion Associated with the Wet Storage of Subsea Pipelines (Wet Parking). In T. L. Skovhus, D. Enning, & J. S. Lee (Eds.), Microbiologically Influenced Corrosion in the Upstream Oil and Gas Industry (1 ed., pp. 361-378). Boca Raton: CRC Press.
- Tuck, B., Watkin, E., Somers, A. Machuca L.L. A Critical Review of Marine Biofilms on Metallic Materials. npj Mater Degrad 6, 25. 2022
- Energy Institute (2017). Guidelines for the management of microbiologically influenced corrosion in oil and gas production.
- For a full list of articles by the Author: https://orcid.org/0000-0002-9590-5421